Major Research Interests
My current research interests primarily focus on the Formulation Engineering of Colloids and Soft Solids. The interplay between formulation and processing (i.e. Formulation Engineering) is at the core of my research activities, which currently explore the design and manufacture of simple and complex emulsion and soft solid microstructures and their resulting functionality. Functionalities of interest presently range from specific material properties (e.g. rheological behaviour) to the use of such microstructures for encapsulation and release purposes. Below is a summary of three of my current principle areas of research activity.
Formulation Engineering of Emulsions, Foams and Soft Solids
My research under this theme includes activities on emulsifier-stabilised, particle-stabilised (Pickering) and mixed emulsifier/particle-stabilised simple (O/W and W/O; FIG 1.A and B, respectively)(1),(2),(3) and complex (W1/O/W2; FIG 1.C and D)(4) emulsions and foams (FIG 1.E and F)(5). I have over twelve years of expertise in the area of Pickering stabilisation in O/W (FIG 1.A), W/O (FIG 1.B), and W1/O/W2 (FIG 1.D) emulsions and foams (FIG 1.F). In parallel to this, my research also focuses on approaches for the fabrication of sub-micron solid particles from edible/natural/sustainable sources and the assessment and enhancement of their Pickering functionality. These solid colloidal structures include crystalline lipid particles (FIG 1.A and B), cellulose-derived particles (FIG 1.D), protein particles (FIG 1.F) and others.
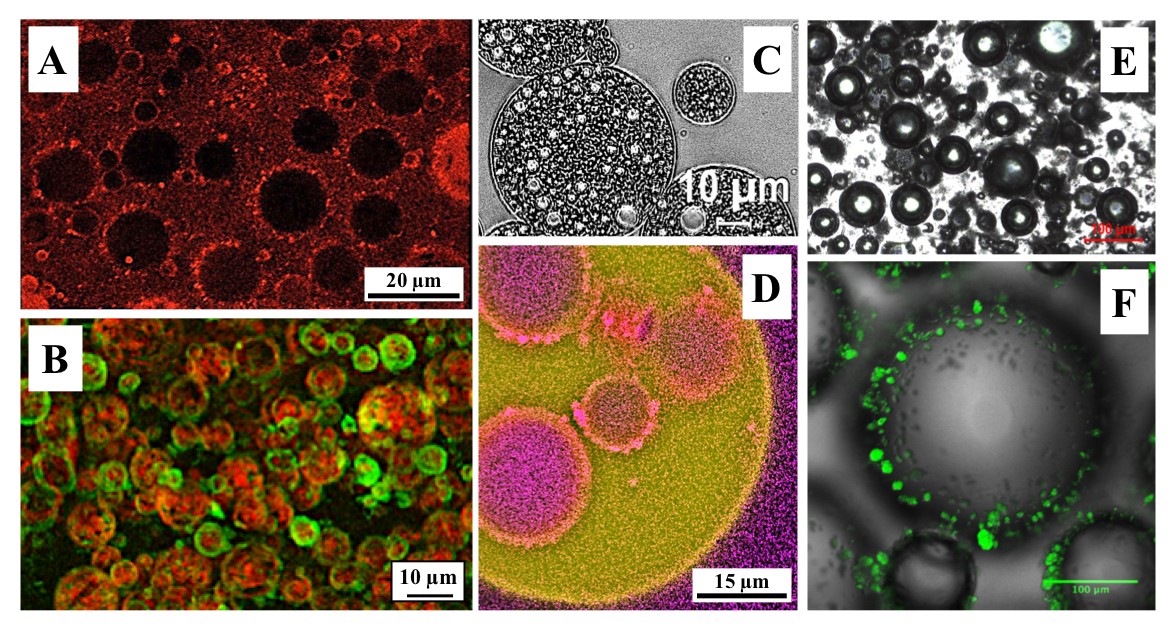
FIGURE 1. A. Confocal micrograph of an O/W emulsion stabilised by wax (cetyl palmitate) particles; fluorescence emission (red) corresponding to the wax particles. B. Confocal micrograph of a W/O emulsion stabilised by mixed mono/triglyceride solid lipid nanoparticles (SLNs); combined fluorescence emissions corresponding to SLNs (green) and water droplets (red). C. W1/O/W2 emulsion produced in a confined impinging jet (CIJ) device (FIG 4.A) and stabilised by PGPR (at the primary W1/O interface) and Tween 20 (at the secondary O/W2 interface). D. W1/O/W2 Pickering emulsion stabilised by ethylcellulose and rutin hydrate particles at the primary and secondary interface, respectively; combined fluorescence emissions corresponding to water (purple) and oil (yellow). E. Light micrograph of a typical foam microstructure. F. Air cells in a foam microstructure stabilised by whey protein isolate (WPI) fluid gel particles.
My research on biopolymeric soft solids spans thirteen years of activities on phase separation phenomena, and interactions in and material properties of single and mixed biopolymer aqueous mixtures. The development, manufacture and functionality of (fluid) gel particles has been one of my main research topics in this area.(6),(7) Fluid gels are (concentrated) suspensions of gel particles in a continuous medium (usually water) that are produced by applying a shear environment (via industrially feasible shear/mixing methods) to a (bio)polymer solution undergoing ionic or thermal gelation. Our group has been studying fluid gels produced by a range of gelling biopolymers (e.g. k-Carrageenan, FIG 2.A)(6); Ca-Alginate, FIG 2.B)(7), at varying polymer concentrations and of different resulting dimensions (FIG 2.C)(7). We have also been investigating the rheological performance(6) of these systems (FIG 2.D), their capacity to be used as encapsulation vehicles(7), as well as whether any such functionalities can be maintained following freeze-drying of these structures and their subsequent reconstitution in aqueous media (FIG 2.D).(7)
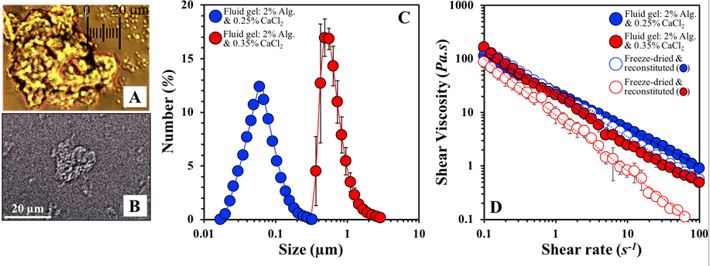
FIGURE 2. A. Light micrograph of a kappa-Carrageenan fluid gel particle. B. Light micrograph of an Alginate/CaCl2 fluid gel particle. C. Size distributions of Ca-Alginate fluid gel particles as a function of CaCl2 concentration. D. Shear viscosities of aqueous dispersions of Ca-Alginate fluid gel particles (same as in FIG 2.C) before and after freeze-drying and reconstitution to their original water content.
Understanding and Development of Emulsification Processes
My research under this theme currently focuses on novel emulsification processes with low energy input and explores their potential industrial applicability. Our group is working in the area of Rotating Membrane Emulsification (RME; inset FIG 3.A),(8),(9),(10) and currently focuses on approaches to enhance the product throughput associated with this process (FIG 3.A) and strategies to induce further reduction to the emulsion droplet sizes that can be achieved (FIG 3.B). We have begun to demonstrate that this is possible by manipulating the hydrodynamic conditions of the continuous (droplet-accepting) phase during RME operation by introducing stationary baffles near the membrane surface. The presence of the baffles has been shown to alter the mixing conditions in the continuous phase. The laminar flow patterns exhibited in the absence of baffles give way to greater recirculation across the system (when at least 4 baffles are put in place) and higher shear forces at the membrane surface where droplet detachment occurs (insets (i), (ii) and (iii) in FIG 3). We now move towards considering baffle positioning and designing baffle geometries (which can be 3D printed) to optimise hydrodynamic conditions so that RME operation is further enhanced.
The group’s research also studies emulsification via confined impinging jet (CIJ) devices (FIG 4.A). Presently, we are working towards fully understanding the turbulent mixing induced within the impingement zone of these devices (FIG 4.A-C) and can process O/W emulsions with up to an 80% oil content.(11) These CIJ apparatuses can be 3D printed and we are currently investigating changes to their geometric features and their impact on the emulsification performance of the device. More recently we have also studied the utilisation of CIJs for the production of more complex emulsion microstructures (double emulsions; FIG 1.C) and fluid gel particles.
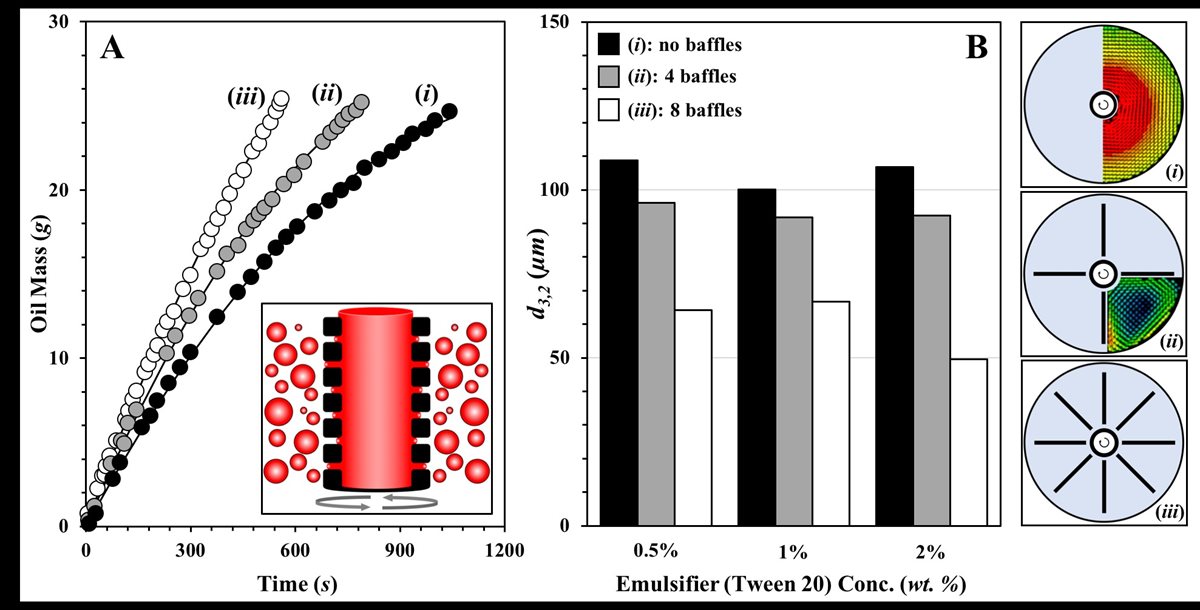
FIGURE 3. A. Dispersed (oil) phase mass introduced (in the form of droplets) in the aqueous continuous phase as a function of rotating membrane emulsification processing time; in each case the slope of the curve is the achieved dispersed phase flux (g/s). B. Emulsion droplet mean diameter (d3,2) as a function of emulsifier (Tween 20) concentration. Inset schematics showing baffle arrangements used during rotating membrane emulsification; (i) no baffles, (ii) 4 baffles, and (iii) 8 baffles. Also shown are spatial velocity fields obtained from Particle Image Velocimetry (PIV) measurements. The PIV study was carried out for a membrane rotating at a speed of 2000 rpm within a vessel containing the continuous phase (water) alone; velocity vectors were acquired for: (i) no baffles and (ii) 4 baffles.
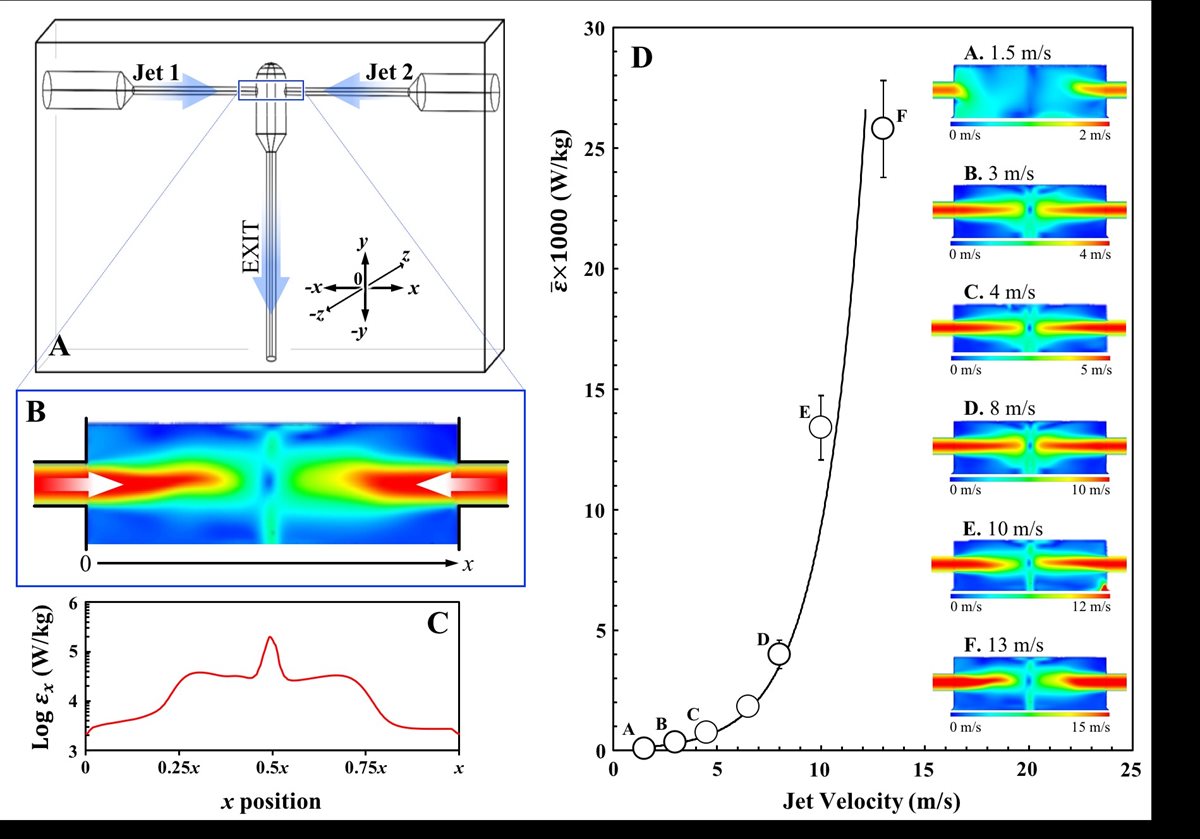
FIGURE 4. An example of a Confined Impinging Jet (CIJ) geometry (A) showing the two channels of jet entry and the exit outlet. A typical CFD velocity profile showing jet collision (B) and associated energy dissipation values () calculated along the jet collision path (x position; 0 ® x) by the model (C). CFD derived mean energy dissipation values () generated within the CIJ chamber as a function of jet velocity (D); inset CFD profiles are also provided for selected jet velocities.
Encapsulation and Release
My research under this theme includes activities on the development of simple and complex assemblies for the encapsulation and controlled delivery of actives; microstructures investigated include simple(12),(13) and double(4) emulsions, nanoemulsions(14), biopolymer sub-micron particles(15), and gelled microparticles(7). We have demonstrated that W/O emulsions, stabilised by lipid particles, can retain salt within their dispersed aqueous droplets (FIG 5.A-C). The droplet size of the emulsions formed following an in situ interfacial crystallization approach (crystallization and emulsification taking place in parallel) is not affected by the presence of salt (FIG 5.A). We have shown that lipid particles form a robust interfacial layer (shell) around the water droplets (FIG 5.B) and significantly limit the release of salt (FIG 5.C). These W/O emulsions can be additionally incorporated within double emulsion microstructures (W1/O/W2) which, depending on the stabiliser used at the O/W2 interface, can exhibit long term stability and consequently assist in further retarding salt release (FIG 5.D).
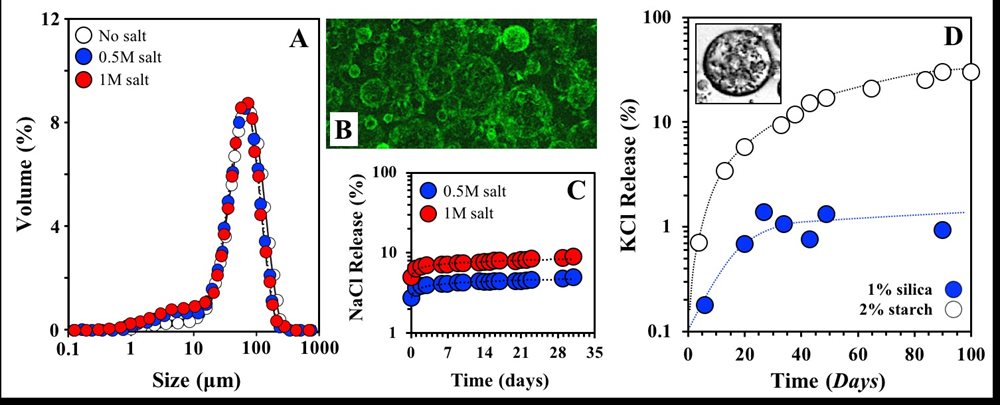
FIGURE 5. A. Size distributions of W/O emulsions with water droplets of varying salt (NaCl) content that are stabilised by lipid particles (shells). B. Confocal micrograph of the lipid particle-stabilised O/W emulsion in FIG 5.A; fluorescence emission (green) corresponding to the lipid particles. C. Salt (NaCl) release from the W/O emulsions in FIG 5.A. D. Salt (KCl) release from the W1/O/W2 emulsions. Primary (W1/O) emulsion (KCl contained within the W1 droplets) stabilised by lipid particles as in FIG 5.A and B, and secondary (O/W2) emulsion stabilised by starch or silica particles.
More recently, my research is focusing on approaches to facilitate the co-encapsulation and co-release of multiple actives from simple emulsion formulations. I have developed a microstructural approach that enables simple O/W and W/O emulsions to be used for the co-encapsulation of two incompatible model actives (FIG 6); one active associated with Pickering particles (FIG 6.A) that are also then used for the stabilisation of emulsion droplets, which themselves contain a second active (FIG 6.B).(13) The co-encapsulation of actives in such a segregated manner has been proven useful for the co-delivery of incompatible species (including components of markedly different hydrophilic/hydrophobic characteristics). Such a co-encapsulation/co-delivery strategy is also beneficial when co-release (from within the same liquid formulation) is required over different timescales and/or in response to external and potentially disparate stimuli/triggers (e.g. pH, magnetic field, mechanical stress). Applied in O/W emulsions (FIG 6.C), this approach enables the co-encapsulation of one hydrophilic (within the biopolymer particles) and a hydrophobic (within the oil droplets) model active and their independent co-release; the first exhibiting a pH-triggered release while the latter is delivered in a sustained fashion that is unaffected by the pH environment. The strategy has also been attempted in W/O emulsions (FIG 6.D), where again the co-encapsulation of one hydrophilic (within the water droplets) and one hydrophobic (within the solid lipid nanoparticles) model active results in their independent co-delivery taking place over significantly disperate timescales.
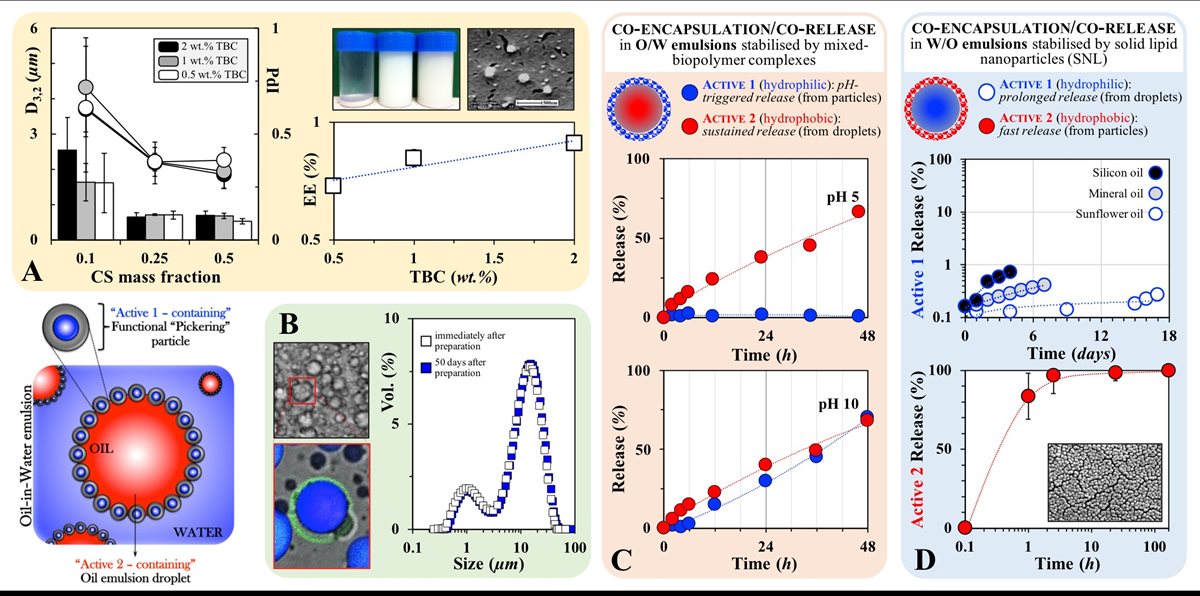
FIGURE 6. A. Mean diameter (bars) and polydispersity index (circles) for sodium caseinate/chitosan (NaCAS/CS) complexes of varying total biopolymer concentration (TBC) and CS mass fractions (mass ratio of chitosan over NaCAS/CS complex). Also shown, encapsulation efficiency (EE) of a hydrophilic model active within the complexes as a function of TBC. Inset images: (left) visual appearance of aqueous suspensions of NaCAS/CS complexes at CS mass fractions (from left to right) of 0.1, 0.25 and 0.5 one week following formation, and (right) cryo-SEM micrograph of the complexes after sonication. B. Optical and confocal micrographs of an O/W emulsion stabilised by the complexes; fluorescence emission correspond to the active enclosed in the complexes (green) and the active contained within the oil droplets (blue). Also shown, size distributions of an O/W emulsion stabilised by complexes. C. Co-Release profiles as a function of pH for Active 1 (hydrophilic) and Active 2 (hydrophobic) from O/W emulsions stabilised by complexes. D. Co-Release profiles for Active 1 (hydrophilic) and Active 2 (hydrophobic) from W/O emulsions stabilised by SNLs.
References
- Zafeiri I., Smith P., Norton I.T., Spyropoulos F., 2017. Fabrication, characterisation and stability of oil-in-water emulsions stabilised by solid lipid particles: The role of particle characteristics and emulsion microstructure upon Pickering functionality. Food Funct., 8(7), 2583.
- Duffus L.J., Norton J.E., Smith P., Norton I.T., Spyropoulos F., 2016. A comparative study on the capacity of a range of food-grade particles to form stable O/W and W/O Pickering emulsions. J. Colloid Interface Sci., 473, 9.
- Pichot R., Spyropoulos F., Norton I.T., 2010. O/W Emulsions stabilised by both low molecular weight surfactants and colloidal particles: The Effect of Surfactant Type and Concentration. J. Colloid Interface Sci., 352(1), 128.
- Frasch-Melnik S., Spyropoulos F., Norton I.T., 2010. W1/O/W2 double emulsions stabilised by fat crystals – Formulation, stability and salt release. J. Colloid Interface Sci., 350(1), 178.
- Lazidis A., Hancocks R.D., Spyropoulos F., Kreuß M., Berrocal R., Norton I.T., 2016. Whey protein fluid gels for the stabilisation of foams. Food Hydrocolloids, 53, 209.
- Gabriele A., Spyropoulos F., Norton I.T., 2009. Kinetic study of fluid gel formation and viscoelastic response with kappa-carrageenan. Food Hydrocolloid, 23(8), 2054.
- Smaniotto F., Zafeiri I., Prosapio V., Spyropoulos F., 2019. Use of alginate fluid gel microparticles to modulate the release of hydrophobic actives. Chem. Eng. Trans., 74, 1219.
- Arkoumanis P.G., Norton I.T., Spyropoulos F., 2019. Pickering particle and emulsifier co-stabilised emulsions produced via rotating membrane emulsification. Colloids Surf A Physicochem Eng Asp., 568, 481.
- Lloyd D.M., Norton I.T., Spyropoulos F., 2015. Process optimisation of rotating membrane emulsification through the study of surfactant dispersions. J. Food Eng., 166, 316.
- Spyropoulos F., Lloyd D.M., Hancocks R.D., Pawlik A.K., 2014. Advances in membrane emulsification. Part A: recent developments in processing aspects and microstructural design approaches. J. Sci. Food Agr., 94(4), 613.
- Tripodi E., Lazidis A., Norton I.T., Spyropoulos F., 2019. On the production of oil-in-water emulsions with varying dispersed phase content using Confined Impinging Jet Mixers. Ind Eng Chem Res.
- Frasch-Melnik S., Norton I.T., Spyropoulos F., 2010. Fat-crystal stabilised w/o emulsions for controlled salt release. J. Food Eng., 98(4), 437.
- Spyropoulos F., Kurukji D., Taylor P., Norton I.T., 2018. Fabrication and Utilization of Bi-functional Protein/Polysaccharide Co-precipitates for the Independent Co-delivery of Two Model Actives from Simple Oil-in-Water Emulsions. Langmuir, 34(13), 3934.
- Pérez-Córdoba L.J., Norton I.T., Batchelor H.K., Gkatzionis K., Spyropoulos F., Sobral P.J.A., 2018. Physico-chemical, antimicrobial and antioxidant properties of gelatin-chitosan based films loaded with nanoemulsions encapsulating active compounds. Food Hydrocolloids, 79, 544.
- Kurukji D., Norton I.T., Spyropoulos F., 2016. Fabrication of sub-micron protein-chitosan electrostatic complexes for encapsulation and pH-Modulated delivery of model hydrophilic active compounds. Food Hydrocolloids, 53, 249-260.